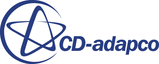
Catalog excerpts
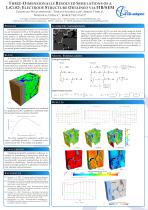
THREE-DIMENSIONALLY RESOLVED SIMULATIONS OF A L1C0O2 ELECTRODE STRUCTURE OBTAINED VIA FIB/SEM CHRISTIAN WALCHSHOFER1, TOBIAS HUTZENLAUB2, SIMON THIELE2, 1CD-adapco (christian.walchshofer@cd-adapco.com), 3 Battery Design LLC (rspotnitz@batdesign.com) 2 Department of Microsystems Engineering - IMTEK, Laboratory for MEMS Applications (tobias.hutzenlaub@hsg-imit.de) Established simulation models for Li-ion batter- ies are formulated in OD or ID (Dualfoil), and rely on assumptions, e.g. well defined particle shapes and absence of diffusion between such particles. Fitted solid conductivities have to be applied to match experiments. The 3D Micro-Structural Elec- trochemistry model implemented in STAR-CCM+ extends the well known Dualfoil model to 3D [1] to accurately predict spatial phenomena. GEOMETRY GENERATION The image stack provided by [2] was read into Scilab using the Scilab Image Processing toolbox (SIP), and coarsened (2x) by a majority wins algorithm. Small regions of minor impact were removed by applying a smoothing algorithm. A mesh was generated using pro-STAR and im- ported into STAR-CCM+. No transport was assumed between binder and electrolyte phases. A Java-macro was used therefore to remove electrolyte regions purely contacting binder and vice-versa, thereby re- moving 0.390% of electrolyte and 0.015% of binder cells. A VARTA LIC 18650 WC lithium-ion battery was segmented by FIB-SEM in [2] and recon- structed (right box). The reconstructed porous elec- trode block was contacted with a current collector, separator and negative foil (carbon) to form a carte- sian mesh simulation setup comprising 21 M cells. Detailed model parametrization was conducted by applying L1C0O2 equilibrium potentials from [3] and electrolyte parameters from [4]. The setup assumed an aluminum current col- lector, a perfectly porous (e = 1) separator and a carbon anode foil. A 1C charge was applied. Modeling electrically conductive pathways ac- curately by introducing a third phase, which rep- resents conductive aid and binder, allows for us- ing physically measured conductivities for active materials in simulations. The third modeled phase leads to stronger gradients in electrolyte concentra- tion, affecting cell performance. [1] Spotnitz et al. 2012 Geometry-resolved electro-chemistry model of li-ion batteries SAE Technical Paper 2012-01-0663 [2] Hutzenlaub et al. 2012 Three-Dimensional Reconstruction of a LiCo02 Li-Ion Battery Cathode Electrochemical and Solid- [3] Karthikeyan, Sikha, White, 2008 Thermodynamic model development for lithium intercalation electrodes. Journal of [4] Gering, K. L. 2006 Prediction of electrolyte viscosity for aqueous and non-aqueous systems: Results from a molec- ular model based on ion solvation and a chemical physics [5] Hutzenlaub et al. 2013 Electrochemical modelling in a FIB /SEM based three-phase reconstruction of a LiCo02 Li- ion battery cathode to appear MODEL FORMULATION Transport equations .DVc-dS- / —=-tdV Butler-Volmer kinetics Charge Curve Cathode Lithiation Range SEI Kinetic Overpotential Range —Maximum —Minimum —Minimum—Maximum—Surface Average Electrolyte Salt Concentration Range —Maximum —Minimum
Open the catalog to page 1All CD-adapco catalogs and technical brochures
-
Dynamics
24 Pages
-
Internal Combustion Engine
20 Pages